Aerodynamics
Boundary layer flows
Experimental Investigation of Boundary Layer Flow over Flat Plate
Turbulent boundary layer over a flat plate is one of the recognized branch of wall bounded shear flow investigation. The monumental contribution of boundary layer research in present day industrial applications, such as aviation and high speed mechanical transport, has added a magnificent magnitude of excellence in creating efficient aerodynamic structures. Nevertheless, at high Reynolds number flow, significant amount of surface friction drag within incompressible domain, constitute 50 % of total drag for aeroplanes [Kornilov et. al. (2005)] and 30 % of total drag for automobiles [Zanoun et. al. (2014)]. To an extended effort, boundary layer investigations are carried out at the Department of Aeodynamics and Fluid Mechanics. Progressive investigations here aims to answer some of the canonical questions of drag reduction mechanism with the help of experimental techniques such as Laser Doppler Anemometry (LDA) and Particle Image Velocimetry (PIV). The Göttingen type wind tunnel used for the boundary layer flow investigation can produce flow at Reynolds number up to 50 m/s with a very reduced amount of turbulence [PhD thesis, V. Motuz (2014)]. Key parameters for this fully developed turbulent boundary layer flow is considered as incompressible. Isothermal condition and zero pressure gradient through out the measurement is assumed.
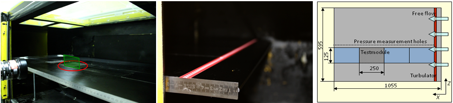
Test section at the tunnel has a dimension of 1.8x0.6.0.5m3 (Figure-1 Left), Flat plate used for the measurement has a dimension of 1.055x0.595m2 (Figure-1 Right). A 'x' tape is used to induce turbulence at the characteristics length of x=0.05m (Figure-1 Center). LDA measurement and PIV flow visualisation were carried out at x=0.615m, LDA measurement location with a red circle and PIV flow field with a green rectangle is indicated respectively (Figure-1 Left). The wall normal and streamwise velocity component has been obtained quantitatively and statisticaly along the wall-normal positions. To determine wall friction, accurate near wall measurement is a very important criteria [Kasagi and Fukagata (2006)]. Moreover, the primary objective with our experiment was to develope a methodology that can obtain higher accuracy within viscous sublayer measurement. With statistically valid data points within the wall normal distance of 12μm to 21μm was achieved with the non intrusive measurement technique (LDA). At a maximum of (where Reɵ represent the Reynolds number based on momentum thickness), mean profile of stream wise component is within y+≤0.6 (Figure-4 Left). Additionally, the turbulent fluctuation in stream wise and wall normal component are in well agreement as a function of wall normal distance (Figure-4 center and right).
Flow structures in their different form, shape and size in wall bounded turbulent flow, impact the amount of surface friction drag [Schlatter et. al. (2014)]. Hence, qualitative and quantitative understanding of the flow is imperative. Literature studies provide us with ample of information stating that beside statistical description of the mean flow, adequate description of the coherent vortical structures are important. From preliminary investigation in this direction with the help of PIV method (Figure-7 Left), existance of coherent structures are ovserved (Figure-7 Center) at . A quantitative representation of an instatntaneous velocity field indicate the existance of vortices at (Figure-7 Right). Continuous effort in developing new experimental methods are on process and published regularly.
Bibliography:
- Kornilov, V.I. , 2005, Reduction of turbulent friction by active and passive methods (Review), Thermophysics and Aeromechanics, Vol. 12, No. 2, P. 175-196.
- Zanoun, E.-S., Jehring, L., Egbers, C. , 2014, Three measuring techniques for assessing the mean wall skin friction in wall-bounded flows, Thermophysics and Aeromechanics, Vol. 21, No. 2, pp 180
- Motuz, V., 2014, Gleichmäßiges Mikro-Ausblasen zur Beeinflussung einer urbulenten Grenzschicht. Doctoral thesis, department of Aerodynamics and Fluid Mechanics, BTU Cottbus-Senftenberg. pp 22
- Kasagi, N., Fukagata, K., 2006, The FIK Identity and Its Implication for Turbulent Skin Friction Control, Transition and Turbulence Control, World Scientific, Singapore, Chapter 10, pp. 297-324.
- Schlatter, P., Li, Q., Örlü, R., Hussain, F., Henningson, D.S., 2014, On the Near-Wall Vortical Structures at Moderate Reynolds Numbers, European Journal of Mechanics B/Fluids, doi:10.1016/j.euromechflu.2014.04.011, pp 75-93
- Hasanuzzaman, G., Merbold, S., Cuvier, C., Motuz, V., Foucaut, J.-M., andEgbers, Ch., (2020). "Experimental investigation of turbulent boundary layers at high Reynolds number with uniform blowing, part I: statistics", Jour. Turb., DOI:https://doi.org/10.1080/14685248.2020.1740239
- Hasanuzzaman, G. Merbold, S., Motuz, V. and Egbers, Ch., (2015). "Experimental Investigation of Building Integrated Ducts with Laser Doppler Anemometry". Laser Methods for Fluid Measurements, German Association for Laser Anemometry (GALA–2015),Dresden Technical University, Dresden, GERMANY, September 08 - 10, pp. 64.1 –6, ISBN 978-3-9816764-1-9, ISSN 2194-2447
link: https://www.gala-ev.org/images/Beitraege/Beitraege%202015/pdf/64.pdf - Hasanuzzaman, G., Merbold, S., Motuz, V. and Egbers, Ch., (2016), "Experimental Investigation of Turbulent Structures and their Control in Boundary Layer Flow", Laser Methods for Fluid Measurements, German Association for Laser Anemometry (GALA–2016), Brandenburg University of Technology, Cottbus, GERMANY, September 06 - 8, DOI: 10.13140/RG.2.2.22847.46247, pp. 27.1 – 6.
- Hassanuzzaman, G., Merbold, S., Motuz, V., Egbers, Ch., (2018), Cuvier, C. and Foucaut,J-M. (2018).Experimental investigation of active control in turbulent boundarylayer using uniform blowing,5th International Conference on Experimental FluidMechanics (ICEFM), 2. - 4. July 2018, pp. 1 – 6.