Fluid Mechanics
Quasi-Biennial-Oscillations (QBO) Experimental study of inertial waves and attractors in a rotating annulus with inner cylinder libration
Inertial waves occur in homogeneous, rotating fluids due to an interplay of Coriolis force and inertia. After excitation they propagate obliquely through the rotating medium. They are relevant for all rotating fluids occurring in nature and industry as well. Many groups studied inertial waves in different geometries. Maas [1] investigated experimentally the propagation of such waves and the generation of a closed inertial ray path, called attractor. The setup used, consisted of a closed rotating tank with rigid lids and an outer side wall sloping down to the middle. The tank was filled with well mixed salt water. Waves were excited due to superimposing a time harmonic oscillation of the tanks rotation rate. Two mechanisms were observed, first the generation of an attractor and second the generation of a strong prograde mean flow originating from the wave reflection point at the sloping wall. With respect to our setup with a time harmonic oscillation of an inner frustum, the following questions need to be addressed:
- What is the mechanism of inertial wave excitation and do we find closed paths of wave energy?
- Can inertial waves drive a mean flow?
Experimental setup
The experiment consists (figure 1a) of a fluid confined between two co-rotating cylinders. Both rotate independently. The outer one is made of borosilicate glass with height 500mm and radius 200mm. The inner one is a frustum, in order to focus wave energy [2, 3] and is made out of aluminum. Its inclination angle is α=5.7°. Rigid lids at the top and bottom close the annular gap. The top lid is made out of acrylic glass and is connected with the outer cylinder. The bottom lid is made out of aluminum, rests on a turntable and serves as support for the outer cylinder
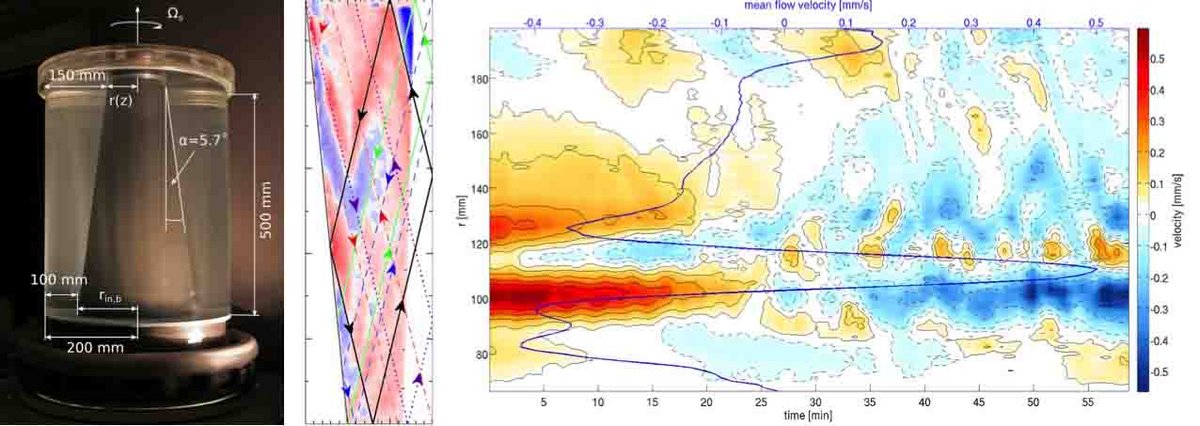
All aluminum parts were anodised to suppress parasitic optical reflections and to make the surface resistant against chemical processes. The apparatus allows mean angular velocities up to Ω0=5.24rad/s. Waves were excited due to super-imposing time harmonic oscillations to the mean angular velocity Ω(t)=Ω0(1+ε·sin[ω·t]) of either the inner frustum or outer cylinder. Here ε is the excitation amplitude adjustable in the interval 0<ε<0.49 and ω is the forcing frequency usually within the inertial wave band 0<ω<2Ω0 [4]. As working fluid we used de-ionised water. Flow visualisations were done with the kalliroscope technique. A camera observes a laser sheet in the radial-axial plane. The fluid is seeded with light reflecting platelets, which align their longest axis parallel to the velocity shear. Viewed from a fix angle the illuminated area exhibits a shading that gives an impression of the streamlines and their evolution with time. By fitting a Fourier-series with the method of least squares we revealed the flow pattern related to the forced frequency (figure 1b). The experiment and the post-processing is described in more detail in [5, 6]. Velocity vector fields are evaluated with the technique of particle image velocimetry (PIV). A radial-azimuthal cross section at heights 100, 200, 300 and 400mm above the bottom lid is observed with a co-rotating camera. The camera acquires the displacement of illuminated hollow-glass spheres (10μm) within two laser pulses. The velocity fields have been computed with the help of the Dantec's DynamicStudio® software package and additionally with MatPIV (Sveen 2004).
Results
Figure 1b shows a least square snapshot in the (r,φ)-plane, resulting from the kalliroscope technique that is plotted underneath the theoretically predicted propagation of wave energy [7]. An 1,1-attractor appears. Color gives light intensity in arbitrary units. Our study revealed that the frequency dependence of the total kinetic energy Kω of the excited wave field is strongly connected to the square of the Ekman pumping velocity wE(ω) that, in the linear limit, becomes singular when the boundary layer erupts and inertial waves are generated in the corners [6].
Also we studied wave-mean flow interaction [8]. An one hour long-time PIV measurement in the co-rotating frame at height 400mm above the lower lid is shown in figure 1c. Waves were excited by libration of the inner frustum at mean rotation rate n=30rpm. The Hovemöller diagram shows the variance of the azimuthal component of v n(t,r). We observe oscillating prograde (red and orange color) as well as a retrograde flows (blueish colors) due to wave-mean flow interaction in a manner qualitatively comparable to the quasi-biennal oscillation (QBO). The time mean azimuthal velocity is shown as thick solid (blue) line. An azimuthal symmetric prograde jet in the interval 100mm<r<120mm appears. It originates at the frustum's bottom corner (here not shown) from non-linear interactions in the Ekman layers and spreads out axially along a surface of constant angular momentum. A second weaker retrograde jet enclosed by the inner frustum and the prograde jet results from angular momentum mixing due to focusing of the reflected inertial wave beam. This feature has been described already by Maas [1].
Bibliography
[1] Maas, L.R.M. (2001) "Wave focusing and ensuing mean flow due to symmetry breaking in rotating fluids" JFM 437, pp. 13-28
[2] Harlander, U., Maas, L.R.M.(2006) "Characteristics and energy rays of equatorially trapped, zonally symmetric internal waves" Meteorologische Zeitschrift 15, pp. 439-450
[3] Maas, L.R.M., Lam, F.P.A. (1995) "Geometric focusing of internal waves." JFM 300, pp. 1-41
[4] Greenspan, H.P. (1990) "The Theory of Rotating Fluids." Breukelen Press, Brookline
[5] Seelig, T. (2014) "Inertial wave propagation, focusing and mean flow excitation: Theory and experiment." Ph.D. Thesis, Cuvillier Verlag Göttingen, 2014.
[6] Klein, M., Seelig, T., Kurgansky, M.V., Ghasemi, A.V., Borcia, I.D., Will, A., Schaller, E., Egbers, Ch., and Harlander, U. "Inertial wave excitation and focusing in a liquid bounded by a frustum and a cylinder. J. Fluid Mech., 751:255-297, 2014. doi:10.1017/jfm.2014.304.
[7] Borcia, I.D. and Harlander, U. (2013) "Inertial waves in a rotating annulus with inclined inner cylinder: comparing the spectrum of wave attractor frequency bands and the eigenspectrum in the limit of zero inclination." Theor. Comput. Fluid Dyn., Vol. 27, issue 3-4, pp. 397-413
[8] Seelig, T. and Harlander, U. "Can zonally symmetric inertial waves drive an oscillating zonal mean flow?" Geophysical & Astrophysical Fluid Dynamics, 109(06):541-566, 2015. doi: 10.1080/03091929.2015.1094064.